The In Vivo Screening Revolution
The importance of in vivo experimentation for disease biology
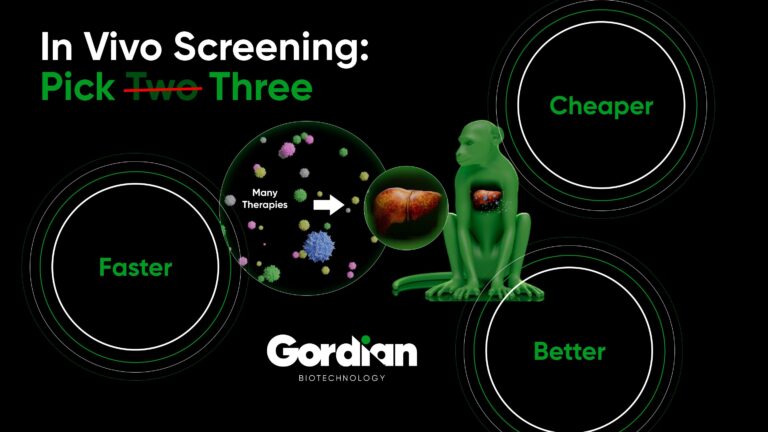
A handful of innovators have developed new, high-throughput in vivo technology that has led the industry to the threshold of a radically different world of drug discovery. This technology will have a game-changing impact on tackling complex and age-related diseases.
Life is a complicated matter. The number of specialized components working in concert to keep an individual cell alive is mind-boggling, let alone the interconnected functioning of the trillions of cells that make up a human. Failures of biology can likewise be complicated. We’ve made great progress in finding solutions to diseases with simple dynamics, such as bacterial infections, largely by scaling up and automating cell culture systems. But this approach has struggled to produce cures for diseases involving more complex dynamics, likely due to the limitations of these ex vivo, cell culture models.
The most conspicuous absence from ex vivo models is a functioning immune system, which is too complex to model ex vivo. For instance, the immune response to cancer cells involves not only the direct attack by cytotoxic T cells but also modulation by regulatory T cells and the influence of cytokines from different cellular sources. This means that a wide range of diseases modeled ex vivo are missing either their natural defense, like clearance of pathogens or tumors, or their primary driver, as in autoimmune destruction of tissue in diabetes or sclerosis.
Immune infiltration and inflammation are also major factors in chronic fibrotic disease.
In e.g. pulmonary and hepatic fibrosis, disease progression is driven by bidirectional feedback loops between fibroblasts and immune cells. Activated macrophages secrete TGF-β and other factors which stimulate fibroblasts to differentiate into myofibroblasts. These myofibroblasts produce extracellular matrix components, leading to tissue scarring. In turn, the extracellular matrix and other factors secreted by myofibroblasts further activate macrophages, perpetuating the cycle of inflammation and fibrosis. This reciprocal interaction is crucial for the development and progression of fibrosis and is difficult to replicate ex vivo, where the dynamic and spatial interactions between these cell types are absent.
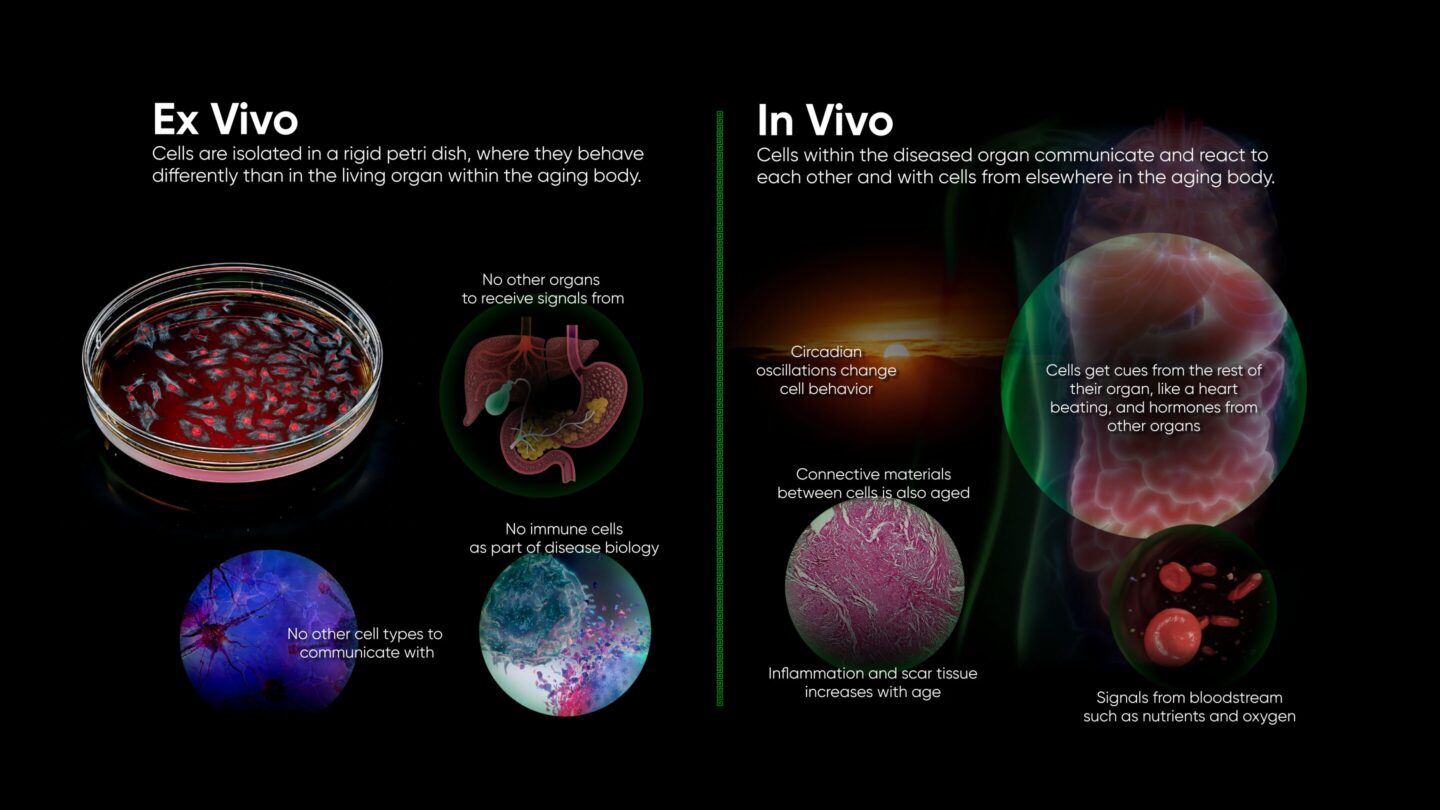
Signals between different cell types are important in every organ, and physical coordination is common as well: synapses between neurons, barriers formed by endo- and epithelium, and heartbeats all rely on physical connections between cells. Electrical, biochemical, and physical signals at the interfaces of cells serve to coordinate cellular actions. Failure of coordination leads to conditions such as atrial fibrillation. Ex vivo models are being developed to replicate such mechanical stressors, but so far, we can only replicate a small local section of an organ.
These are just examples that we know about. New mechanisms and interactions are discovered every year, so it is obvious that we are nowhere near fully understanding physiology. Thus, even the most carefully constructed ex vivo model system risks leaving out aspects of biology we didn’t realize were important.
The reality remains that the only model systems that allow for the inclusion of all relevant known and unknown biology for the disease in question are living diseased animals.
Why does this matter for drug discovery?
Drug discovery aims to identify therapeutics that work in living humans. For obvious reasons, confirming that newly discovered therapeutics are indeed efficacious and safe in humans must be done with the utmost of ethical consideration. This is the reason behind carefully regulated clinical trials. Prior to trials, evidence is built to motivate the expense and risk associated with clinical trials. This preclinical work is split into two central foci:
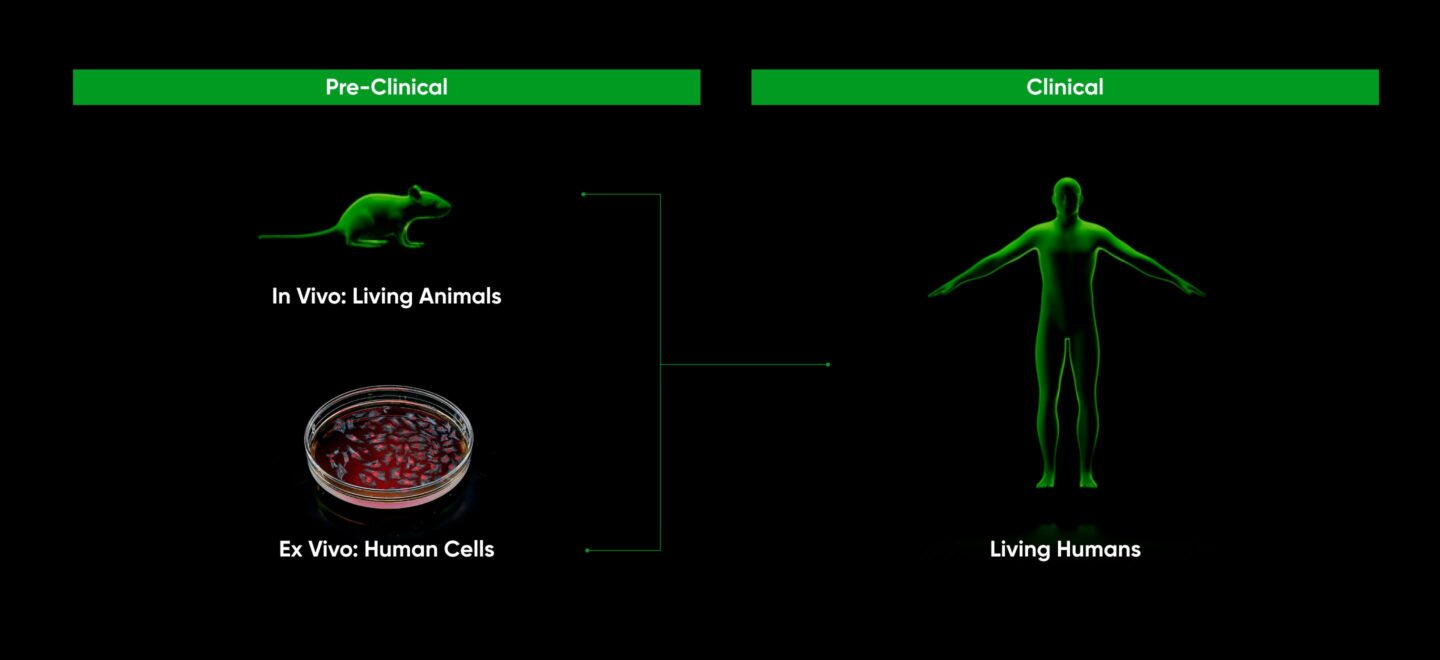
Advances in high-throughput screening have enabled rapid and cheap exploration of therapeutics in human cell lines. Because these cells are easily manipulated outside a living animal, millions of experiments can be run in parallel. But these experiments lack the in vivo environment described above. Thus their results must be validated in a living animal. So far, this validation process has been slow and expensive.
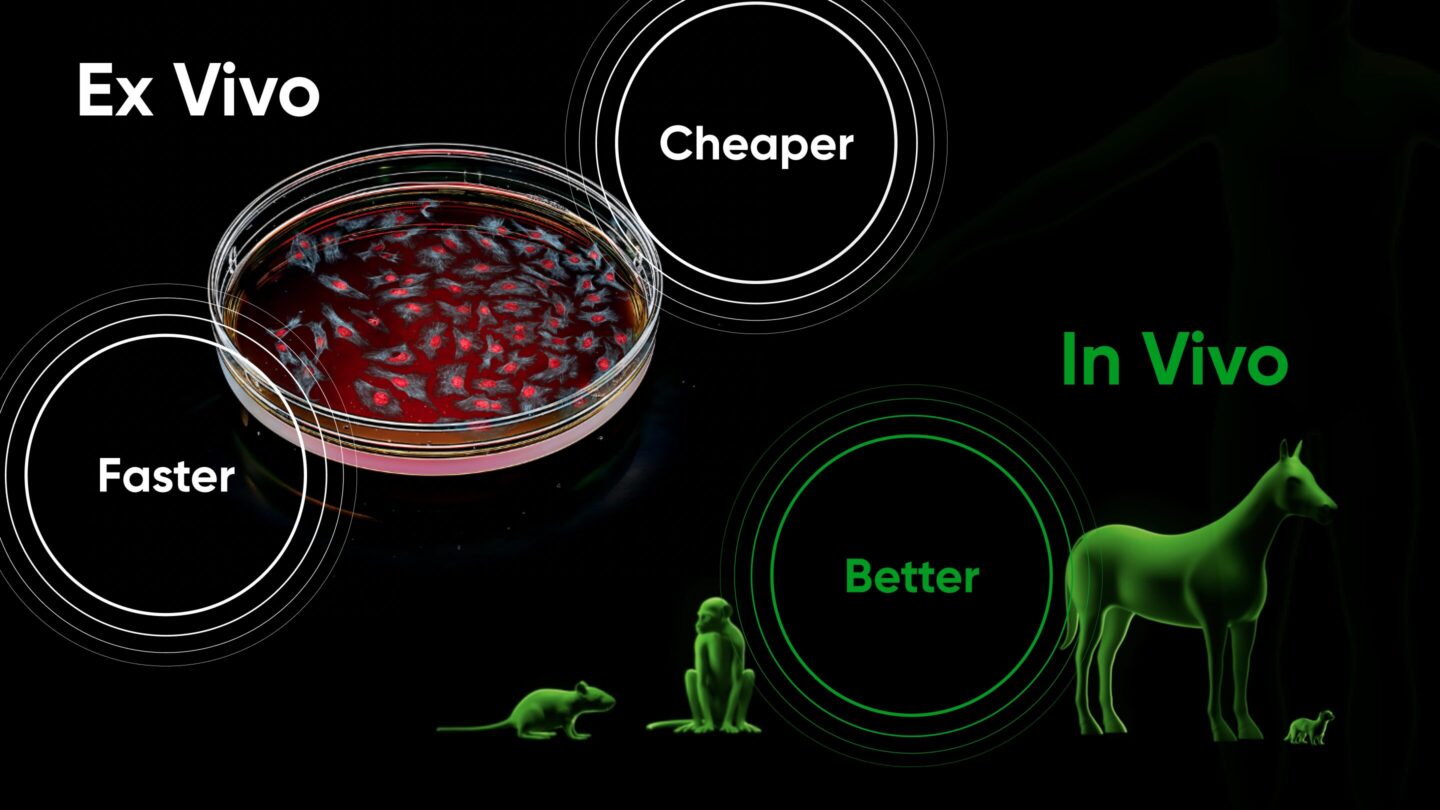
Because of this relationship, ex vivo testing has traditionally taken place first, narrowing the funnel of potential therapeutics going into in vivo testing in order to minimize total cost. The results of in vivo testing are fed back into ex vivo testing, and the loop progresses until a suitable therapeutic is discovered. Drug discovery thus typically progresses along three stages:
- High-throughput screening: Millions of therapies are tested in parallel ex vivo, in order to identify molecules that engage the target.
- Hit to lead: Promising therapies undergo limited optimization ex vivo to enable suitability for in vivo testing.
- Lead optimization: Therapies are further optimized in order to maximize in vivo efficacy. Results of ex vivo optimization experiments are fed into in vivo validation experiments, in order to iteratively arrive at an optimized therapy.
Once discovery is complete, the optimized therapeutic undergoes IND (investigational new drug)-enabling studies and enters clinical trials. The progression of discovery and associated costs per phase are described in S. Paul et al, Nature Reviews, Drug Discovery, vol 9, March 2010 and summarized below:
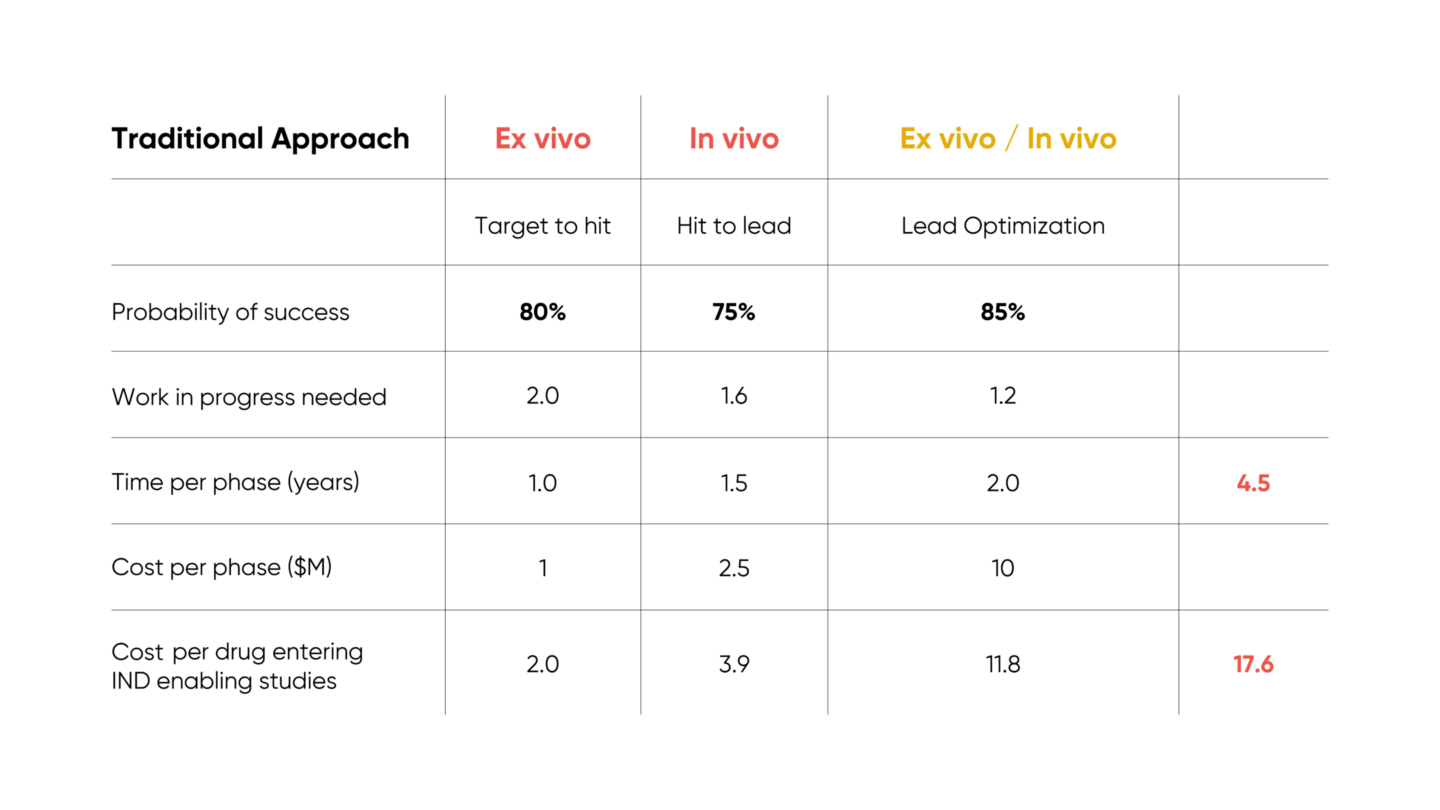
Of note, the loop between cheap ex vivo testing and costly in vivo testing results in significant expense, particularly in the lead optimization phase.
The power of in vivo screening
High throughput in vivo screening ushers in a new paradigm. In this case, millions of experiments can be run in parallel, in the full context of the in vivo biology necessary for ultimately vetting therapeutics for clinical trials. Further, every therapeutic hit is, by definition, functional in vivo and thus requires relatively little optimization post-discovery. There is no ex vivo / in vivo loop, rather everything is complete in vivo in one step. The best of both worlds is achieved.
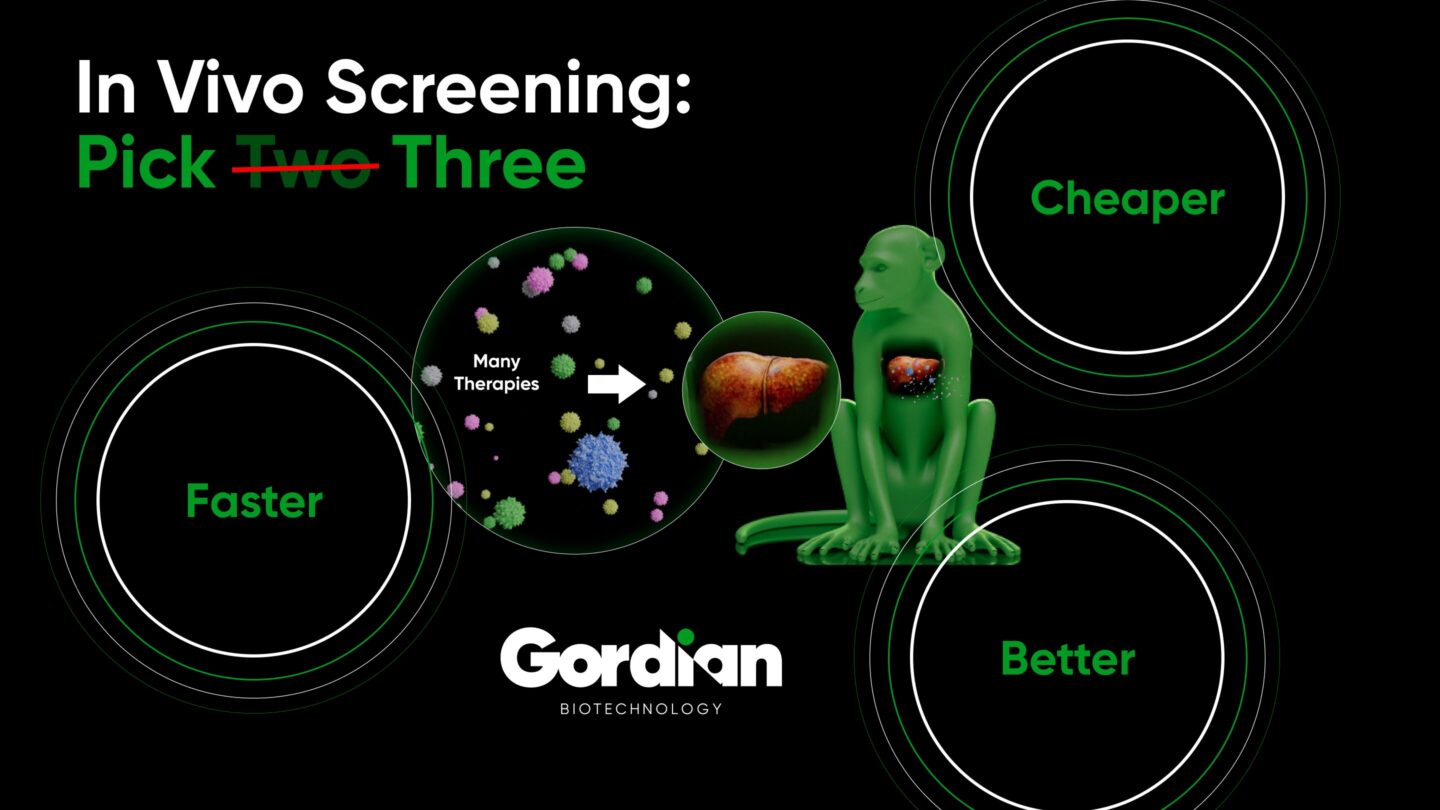
Several companies and institutions are working on enabling high throughput in vivo screening. Gordian’s approach (described in our introductory blog post and in more detail in our white paper) identifies novel therapeutics for complex age-related diseases, and results in over 3x improvement in time required and 10x improvement in cost required per IND-enabling study
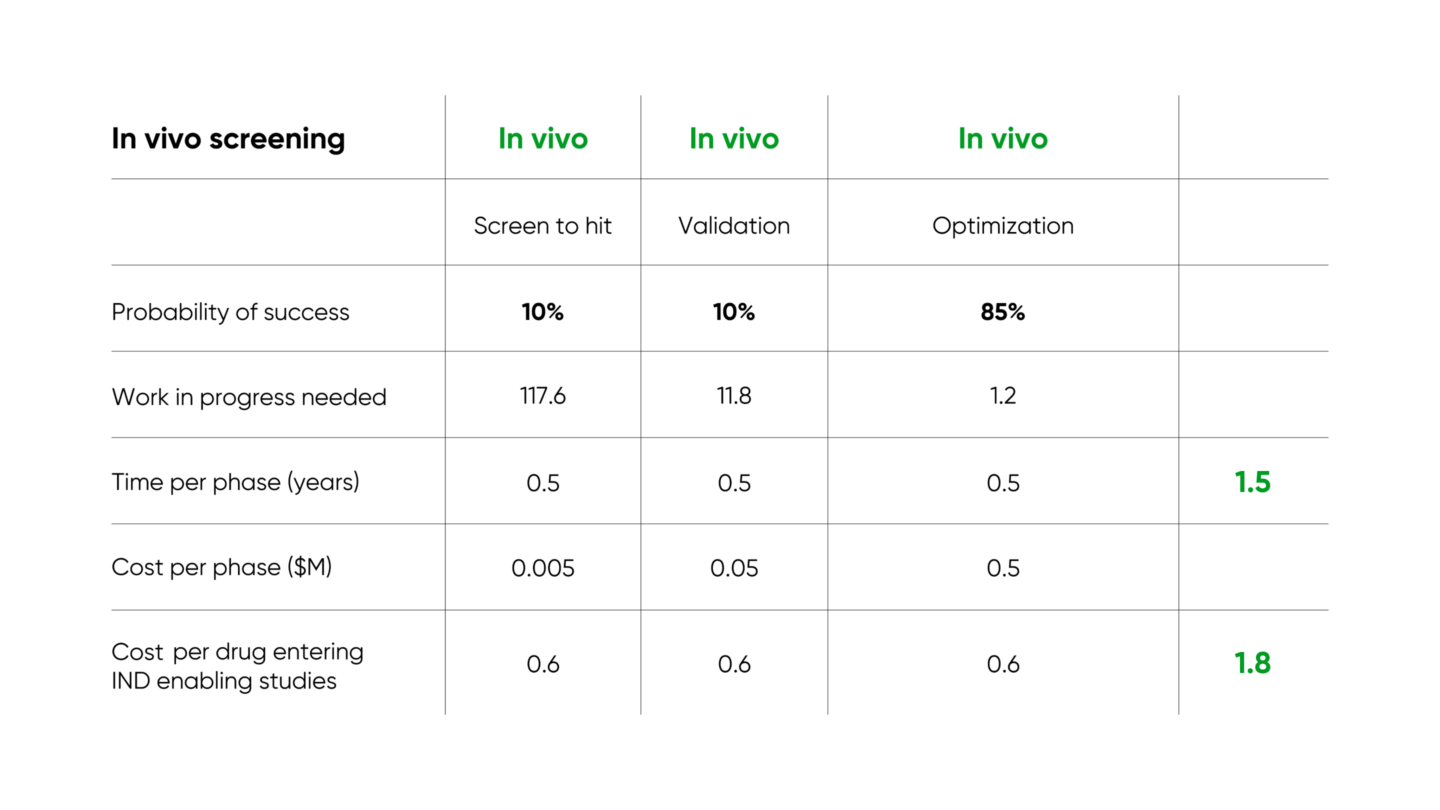
Other companies are also researching pooled in vivo screening for different problems in drug development. Manifold Bio and Waypoint Bio are working on delivering cancer therapies to tumors with high specificity, for protein drugs and cell therapies respectively. Vevo Therapeutics is testing cancer drugs against pools of cells from different patients implanted into living mice, so that test results include mechanisms of drug resistance that span human heterogeneity, and depend on interactions with the cellular environment.
Faster, cheaper, and better: the impact of in vivo screening on clinical development
The cheaper cost and faster timeline of discovery enabled by in vivo screening have a massive impact on drug development as a whole. Because the majority of the cost associated with drug development is in running clinical trials, maximizing the probability of clinical trial success is imperative. High-throughput in vivo screening enables preclinical exploration of orders of magnitude more targets and therapeutics for a given condition. The bar for moving out of preclinical research and into IND-enabling studies and clinical trials can be made much higher, allowing only far superior therapeutics to advance.
Even further, in vivo pooled screening enables the use of more advanced animal models that offer better clinical translation. Most traditional in vivo studies are done in models where disease-like pathology is induced rapidly and artificially: tumors are implanted into non-cancerous tissue, toxins are used to simulate degenerative disease, organs are damaged or removed using surgery. While these accelerated models indeed provide an in vivo test environment, this environment is somewhat artificial, and thus carries many of the deficiencies of ex vivo tests.
On the other hand, using in vivo screening, only a few animals are required to produce data that otherwise would have required thousands of animals. This loosens logistical and financial constraints, enabling the use of (costly) large or advanced animal models that have developed disease progressively and/or spontaneously, much like the patients they are intended to model. For example, Gordian’s Osteoarthritis in vivo screens utilize naturally occurring horse models, rather than the mechanically induced mouse models typically used.
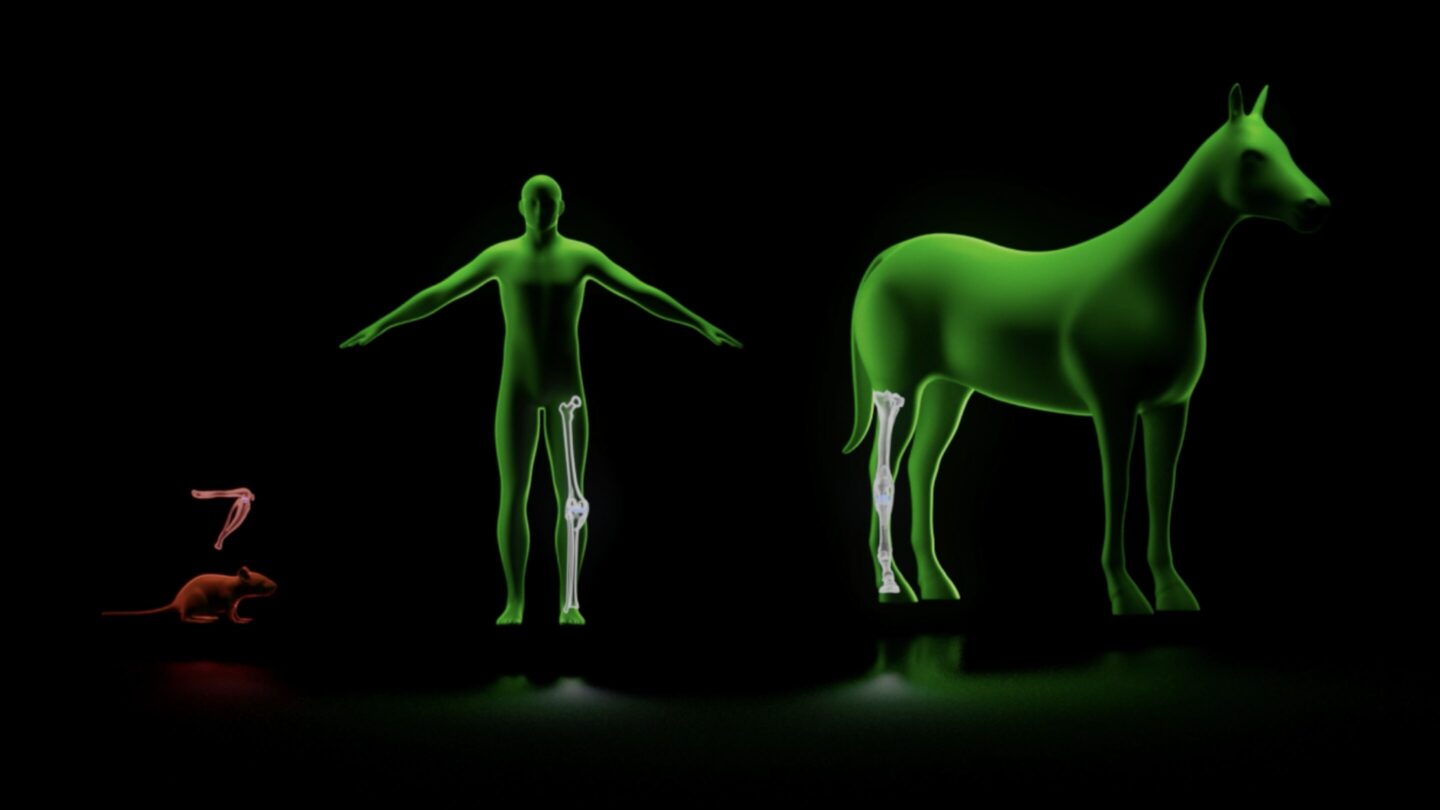
This means in vivo screening enables experiments that were once practically impossible. Screening 100 novel targets in naturally occurring horse models of osteoarthritis would require approximately 1,000 horses using traditional methods (10 horses per intervention cohort). Even disregarding the extremely large expense of such a study (several tens of millions of dollars), there is no institution on the planet that could support such an experiment. On the other hand, generating analogous in vivo data from a high throughput in vivo screen requires two horses, and costs approximately $500K, an experiment Gordian has already performed several times over.
The revolution is here